Global Navigation Satellite Systems (GNSS) have become an indispensable part of modern navigation and positioning applications, and the development of Precise Point Positioning (PPP) technology signifies significant progress in achieving high-precision location information. This article explores the latest research, providing a comparative analysis of typical performance indicators such as PPP float solutions, PPP fixed solutions, positioning accuracy, and initialization time for single-system/multi-system PPP under current satellite constellation conditions.
- Background
Global satellite navigation systems provide high-quality positioning, navigation, and timing services to users worldwide, playing a crucial role in national economic development and military defense. The precision, reliability, and timeliness of satellite navigation directly determine its scientific and engineering applications.
Due to various error sources, the positioning accuracy provided by satellite navigation systems is typically in the range of 5 to 10 meters, insufficient for the needs of high-precision users. To achieve centimeter or even millimeter-level positioning, geodesists have proposed two precise positioning methods: differential positioning and Precise Point Positioning (PPP). PPP, with the ability to simultaneously estimate precise station coordinates, receiver clock errors, absolute tropospheric delays, horizontal gradients, and tilted-path ionospheric delays, offers significant advantages. Therefore, PPP has unique application value in GNSS precise positioning and orbit determination, precise timing, atmospheric science, and Earth dynamics.
- GNSS PPP Float and Fixed Solutions: Results and Analysis
This article utilizes observation data from 53 stations globally distributed from January 1 to January 7, 2020 (Figure 1) to conduct dual-frequency PPP positioning experiments, including single-system and multi-system, static and kinematic, PPP float, and fixed solutions. The aim is to evaluate the static and kinematic positioning performance of current GNSS single-system and multi-system PPP (simulated dynamic) under various satellite constellation conditions. Given the limited support for the BeiDou-3 system in existing analysis centers' precise satellite orbit and clock products, and the fewer MGEX stations supporting BeiDou-3 observations, this study includes 21 iGMAS stations to better assess the BeiDou system's precise point positioning performance.
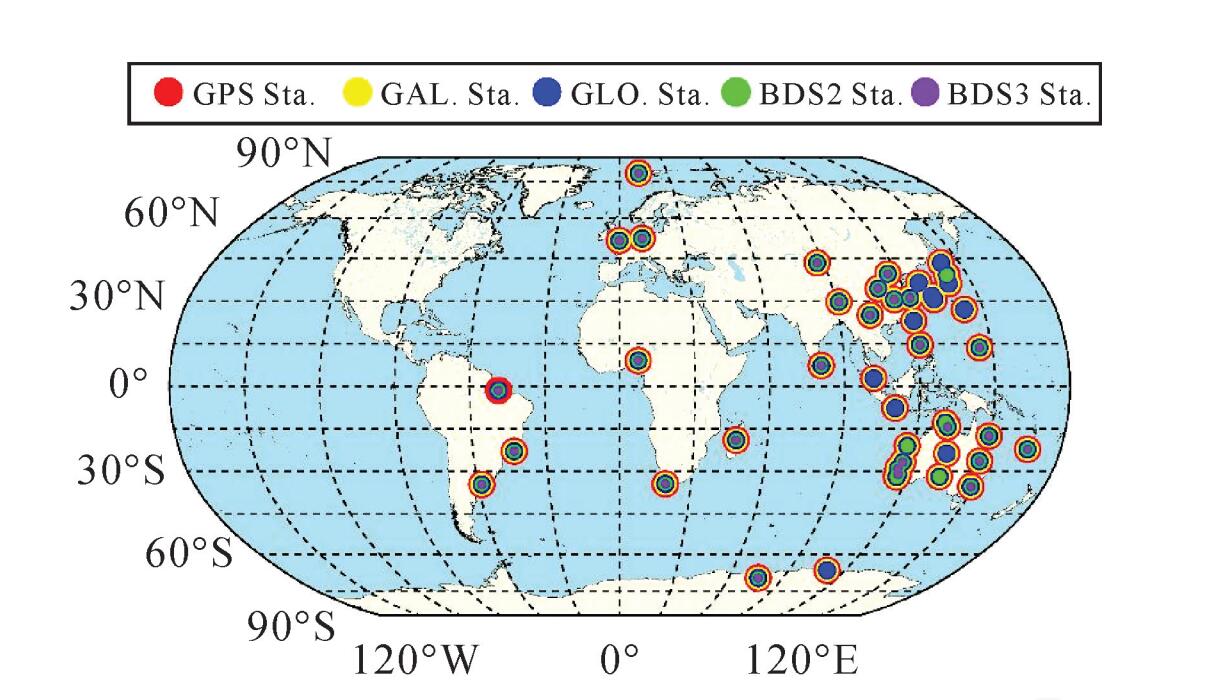
In the data statistics of this article, the following definitions are applied to positioning performance indicators: static solution positioning accuracy is defined as the accuracy at the last epoch of convergence for the day; kinematic solution positioning accuracy is the average accuracy of each epoch after convergence; reference coordinates are obtained from SNX weekly solution files. If reference coordinates are not available in the weekly solution file, the average of the four-system static positioning results for a 7-day PPP solution at the corresponding station is taken. Convergence time is defined as the time required for the three-dimensional positioning error to be less than 1 dm and maintained for at least ten epochs. First fixed time is the time required for the ambiguities to be correctly fixed and the fixed solution accuracy to exceed the float solution accuracy.
2.1 Static Positioning
In static PPP float and fixed solution experiments, this section presents the single-day results for a single station, evaluating static PPP float and fixed solution performance based on positioning accuracy, convergence time, and first fixed time. Figure 2 provides representative static PPP float and fixed solutions for station MRO1 on January 7, 2020, for GPS, Galileo, GLONASS, BDSG2, BDSG2+3, and the combination of these systems. It also includes the daily time series of visible satellite counts for each system during periods when solutions are available.
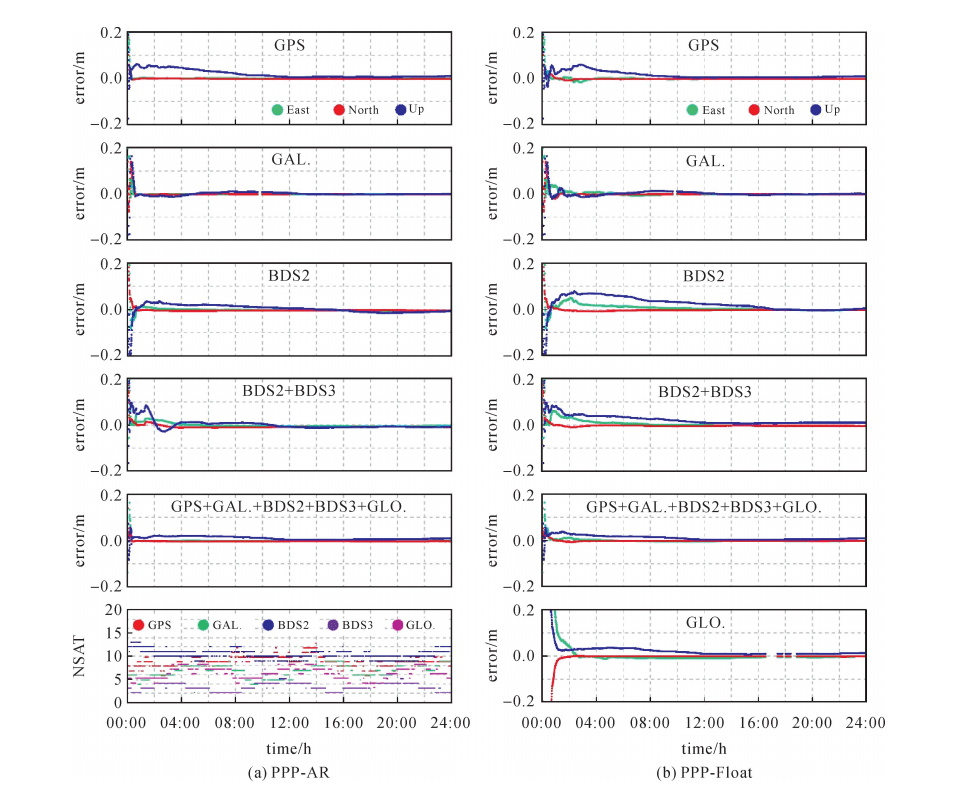
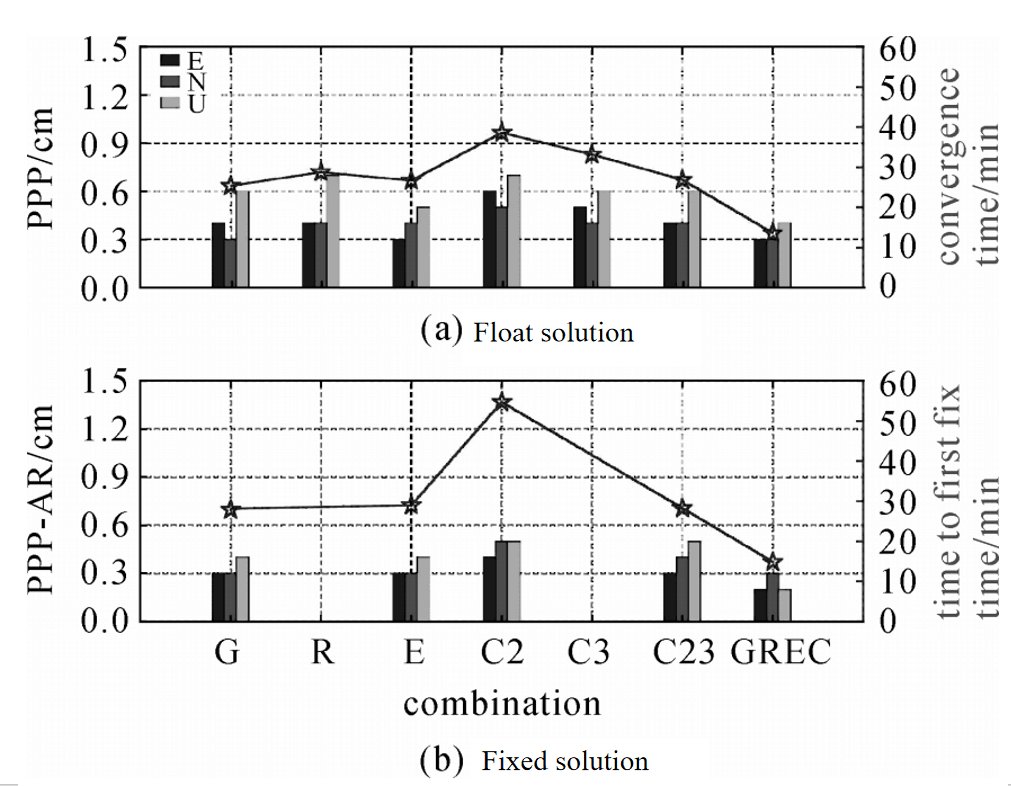
As the selected station cannot optimize the observations and conditions for all satellite systems simultaneously, its results cannot represent the optimal level achievable by each satellite system. Therefore, Figure 3 and Table 1 present statistical results for PPP using 53 stations. For convenience, the letters G, R, E, and C are used to represent GPS, GLONASS, Galileo, and BDS, respectively.
Considering the unfavorable observation conditions for BeiDou-3 system satellites at MGEX stations, the results from iGMAS stations are primarily used for analyzing BeiDou system positioning performance. From the statistical results, it is observed that multi-system combined PPP float solutions exhibit significantly shorter convergence times and first fixed times compared to single systems. The convergence time and first fixed time for multi-system combined PPP float solutions are 13.6 minutes and 14.9 minutes, respectively. For single-system PPP static solutions (G/R/E/C), the post-convergence positioning accuracy is generally at a comparable level, with GPS and Galileo slightly outperforming GLONASS and BeiDou. The positioning accuracy of multi-system combined PPP is superior to single-system PPP.
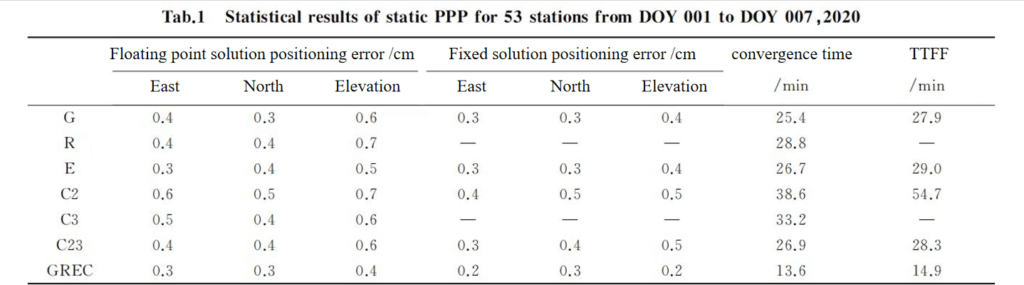
2.2 Simulated Kinematic Positioning
Figure 4 provides representative results for dynamic PPP float and fixed solutions for station MRO1 on January 7, 2020, involving GPS, Galileo, GLONASS, BDSG2, BDSG2+3, and the combination of multiple systems. It also displays the number of visible satellites for each system during periods when solutions are available. The stability of dynamic PPP fixed solutions is evident in comparison to float solutions. In moments of insufficient satellite count, Galileo and GLONASS parameters are reinitialized during the positioning solution. The performance of four-system combined PPP remains significantly superior to single systems, with reduced positioning deviations and notable improvements in positioning accuracy. Effective enhancements in PPP positioning accuracy are observed when ambiguities are correctly fixed and propagated.
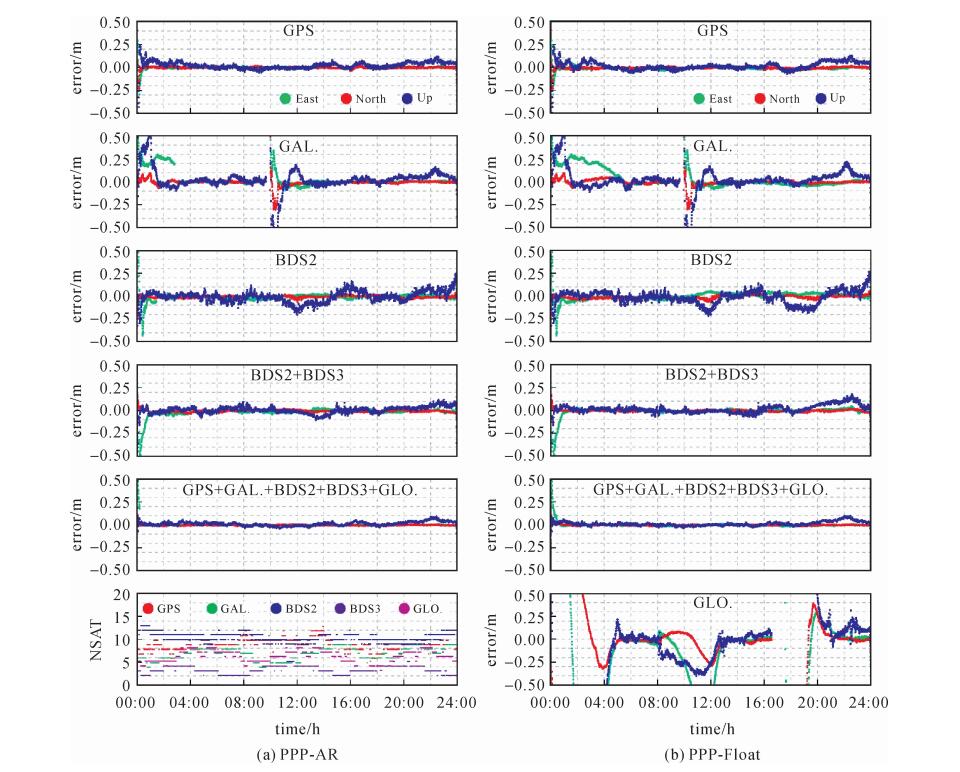
Fig.4 Kinematic PPP ambiguity resolution (a) and float solution (b) at station MRO1 on DOY 007,2020
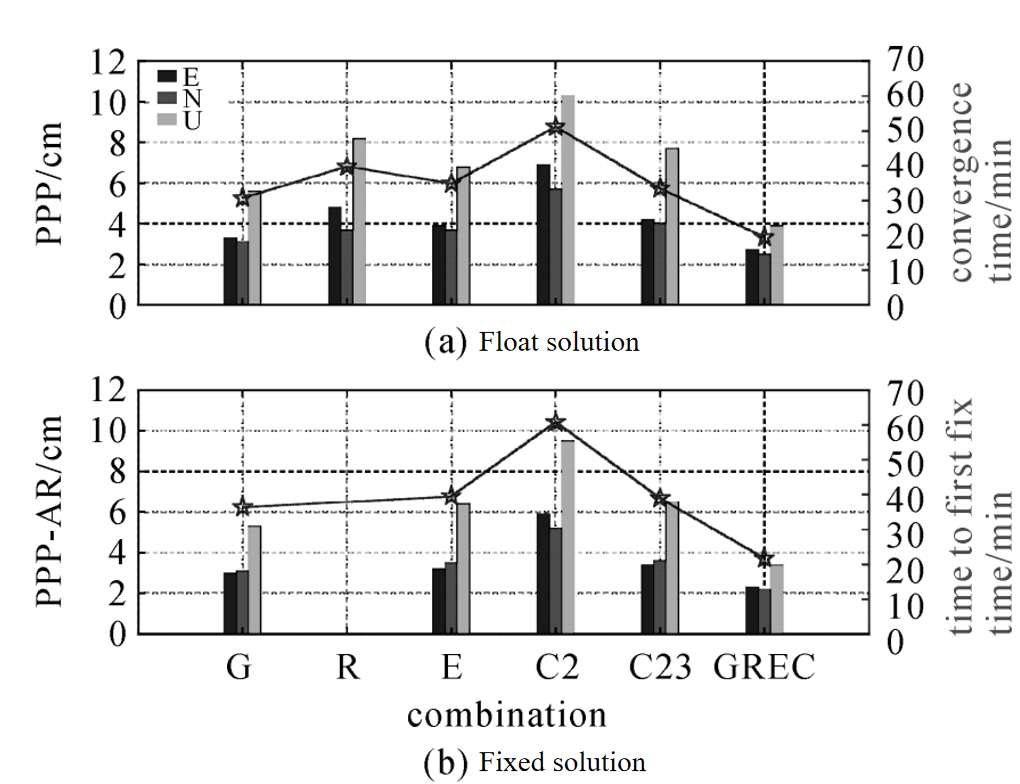
Table 2 and Figure 5 present statistical results for one-week dynamic PPP using 53 stations. It is observed from Table 2 that the convergence time and first fixed time for dynamic PPP are longer than those for static PPP under similar conditions. The positioning accuracy of multi-system combined PPP float solutions is improved by 18.7% horizontally and 30.4% vertically compared to single GPS PPP float solutions.
Upon correct ambiguity fixing, fixed solution positioning accuracy is significantly better than float solution accuracy. Multi-system combined fixed solutions improve positioning accuracy by 14.8%, 12.0%, and 12.8% in the east, north, and up directions, respectively, compared to float solutions. The convergence time and first fixed time for multi-system combined PPP float and fixed solutions are shorter than those for single GPS, with reductions of 36.5% and 40.4%, respectively, indicating noticeable improvements.
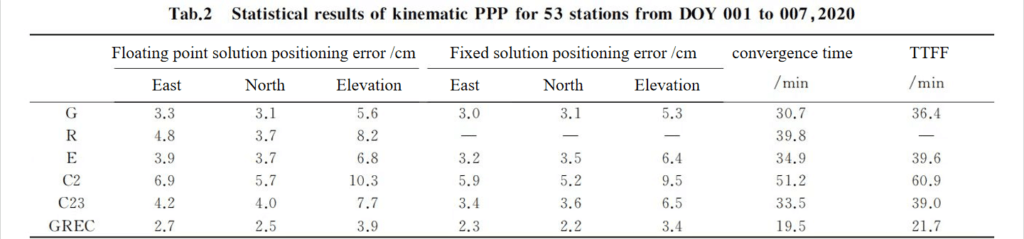
3. Conclusion and Outlook
This article analyzes and discusses the PPP positioning performance of GPS, GLONASS, Galileo, and BDS using the latest multi-system GNSS observational data. Regarding positioning accuracy, both float and fixed solutions for static PPP achieve comparable accuracy levels after convergence, reaching millimeter-level precision. The introduction of multi-system GNSS fusion results in a moderate improvement in positioning accuracy, but the enhancement is not significant. For dynamic PPP, multi-system fusion significantly improves positioning accuracy, with a horizontal and vertical accuracy improvement of 18.7% and 30.4%, respectively, compared to single GPS dynamic PPP float solutions.
Regarding convergence/initialization time, GLONASS and BDS exhibit slightly longer convergence times than GPS and Galileo, with multi-system GNSS combination PPP showing the fastest convergence speed. Compared to single GPS, the convergence time and first fixed time for multi-system combined float solutions are reduced by 36.5% and 40.4%, respectively, indicating significant improvements.
Additionally, if higher positioning accuracy is required, or there are time constraints, users may opt for Real-Time Kinematic (RTK) technology. For example, the ER-GNSS-M10 module supports full-system, full-frequency RTK positioning. However, the accuracy achieved by RTK positioning depends largely on the distance from the base receiver, and increasing the RTK baseline length may reduce accuracy and initialization time. Therefore, the choice between PPP and RTK should be made based on the required accuracy level, time constraints, and the availability of specific devices in different measurement applications. If you have any questions, feel free to contact us at any time.
I will appreciate it if this article helps you a lot. For more information about GNSS, please read the articles below or feel free to contact us:
Email: info@ericcointernational.com
More Technical Questions
1. Precision Mapping in Mining Operations: The Role of GNSS Augmentation Technology
2. Application of GNSS-RTK Positioning Technology in ICV Testing
3. Integration of GNSS Modules in Aviation: Precision Navigation for Unmanned Aerial Vehicles (UAVs)
4. Decoding Navigation Technology: GNSS Boards vs. GNSS Modules – Unveiling the Distinctions
5. Distinctions Between GNSS Receivers and Antennas
6. How to select a GNSS antenna?
Products in Article
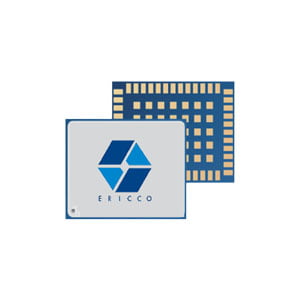
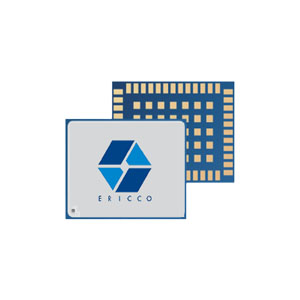
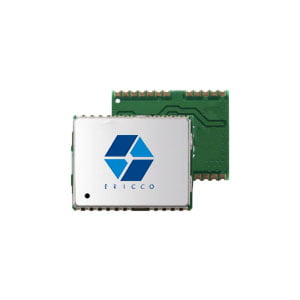
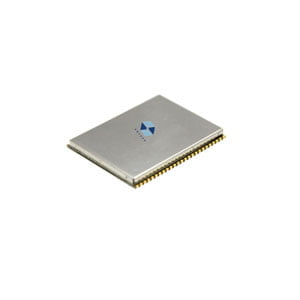
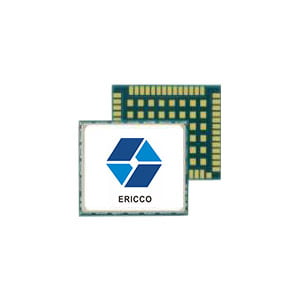
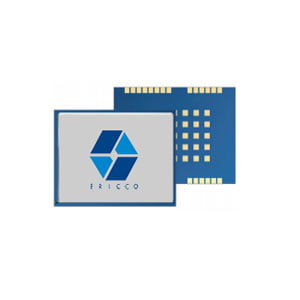